1
The Microgrids Concept
1.1 Introduction
Modern society depends critically on a secure supply of energy. Growing concerns for primary energy availability and aging infrastructure of current electrical transmission and distribution networks are increasingly challenging security, reliability and quality of power supply. Very significant amounts of investment will be required to develop and renew these infrastructures, while the most efficient way to meet social demands is to incorporate innovative solutions, technologies and grid architectures. According to the International Energy Agency, global investments required in the energy sector over the period 2003–2030 are estimated at $16 trillion.
Future electricity grids have to cope with changes in technology, in the values of society, in the environment and in economy [1]. Thus, system security, operation safety, environmental protection, power quality, cost of supply and energy efficiency need to be examined in new ways in response to changing requirements in a liberalized market environment. Technologies should also demonstrate reliability, sustainability and cost effectiveness. The notion of smart grids refers to the evolution of electricity grids. According to the European Technology Platform of Smart Grids [2], a smart grid is an electricity network that can intelligently integrate the actions of all users connected to it – generators, consumers and those that assume both roles – in order to efficiently deliver sustainable, economic and secure electricity supplies. A smart grid employs innovative products and services together with intelligent monitoring, control, communication and self-healing technologies.
It is worth noting that power systems have always been “smart”, especially at the transmission level. The distribution level, however, is now experiencing an evolution that needs more “smartness”, in order to
- facilitate access to distributed generation [3,4] on a high share, based on renewable energy sources (RESs), either self-dispatched or dispatched by local distribution system operators
- enable local energy demand management, interacting with end-users through smart metering systems
- benefit from technologies already applied in transmission grids, such as dynamic control techniques, so as to offer a higher overall level of power security, quality and reliability.
In summary, distribution grids are being transformed from passive to active networks, in the sense that decision-making and control are distributed, and power flows bidirectional. This type of network eases the integration of DG, RES, demand side integration (DSI) and energy storage technologies, and creates opportunities for novel types of equipment and services, all of which would need to conform to common protocols and standards. The main function of an active distribution network is to efficiently link power generation with consumer demands, allowing both to decide how best to operate in real-time. Power flow assessment, voltage control and protection require cost-competitive technologies and new communication systems with information and communication technology (ICT) playing a key role.
The realization of active distribution networks requires the implementation of radically new system concepts. Microgrids [5–11], also characterized as the “building blocks of smart grids”, are perhaps the most promising, novel network structure. The organization of microgrids is based on the control capabilities over the network operation offered by the increasing penetration of distributed generators including microgenerators, such as micro-turbines, fuel cells and photovoltaic (PV) arrays, together with storage devices, such as flywheels, energy capacitors and batteries and controllable (flexible) loads (e.g. electric vehicles [12]), at the distribution level. These control capabilities allow distribution networks, mostly interconnected to the upstream distribution network, to also operate when isolated from the main grid, in case of faults or other external disturbances or disasters, thus increasing the quality of supply. Overall, the implementation of control is the key feature that distinguishes microgrids from distribution networks with distributed generation.
From the customer's point of view, microgrids provide both thermal and electricity needs, and, in addition, enhance local reliability, reduce emissions, improve power quality by supporting voltage and reducing voltage dips, and potentially lower costs of energy supply. From the grid operator's point of view, a microgrid can be regarded as a controlled entity within the power system that can be operated as a single aggregated load or generator and, given attractive remuneration, also as a small source of power or ancillary services supporting the network. Thus, a microgrid is essentially an aggregation concept with participation of both supply-side and demand-side resources in distribution grids. Based on the synergy of local load and local microsource generation, a microgrid could provide a large variety of economic, technical, environmental and social benefits to different stakeholders. In comparison with peer microsource aggregation methods, a microgrid offers maximum flexibility in terms of ownership constitution, allows for global optimization of power system efficiency and appears as the best solution for motivating end-consumers via a common interest platform.
Key economic potential for installing microgeneration at customer premises lies in the opportunity to locally utilize the waste heat from conversion of primary fuel to electricity. There has been significant progress in developing small, kW-scale, combined heat and power (CHP) applications. These systems have been expected to play a very significant role in the microgrids of colder climate countries. On the other hand, PV systems are anticipated to become increasingly popular in countries with sunnier climates. The application of micro-CHP and PV potentially increases the overall efficiency of utilizing primary energy sources and consequently provides substantial environmental gains regarding carbon emissions, which is another critically important benefit in view of the world's efforts to combat climate change.
From the utility point of view, application of microsources can potentially reduce the demand for distribution and transmission facilities. Clearly, distributed generation located close to loads can reduce power flows in transmission and distribution circuits with two important effects: loss-reduction and the ability to potentially substitute for network assets. Furthermore, the presence of generation close to demand could increase service quality seen by end customers. Microgrids can provide network support in times of stress by relieving congestion and aiding restoration after faults.
In the following sections, the microgrid concept is clarified and a clear distinction from the virtual power plant concept is made. Then, the possible internal and external market models and regulation settings for microgrids are discussed. A brief review of control strategies for microgrids is given and a roadmap for microgrid development is provided.
1.2 The Microgrid Concept as a Means to Integrate Distributed Generation
During the past decades, the deployment of distributed generation (DG) has been growing steadily. DGs are connected typically at distribution networks, mainly at medium voltage (MV) and high voltage (HV) level, and these have been designed under the paradigm that consumer loads are passive and power flows only from the substations to the consumers and not in the opposite direction. For this reason, many studies on the interconnection of DGs within distribution networks have been carried out, ranging from control and protection to voltage stability and power quality.
Different microgeneration technologies, such as micro-turbines (MT), photovoltaics (PV), fuel cells (FC) and wind turbines (WT) with a rated power ranging up to 100 kW can be directly connected to the LV networks. These units, typically located at users' sites, have emerged as a promising option to meet growing customer needs for electric power with an emphasis on reliability and power quality, providing different economic, environmental and technical benefits. Clearly, a change of interconnection philosophy is needed to achieve optimal integration of such units.
Most importantly, it has to be recognized that with increased levels of microgeneration penetration, the LV distribution network can no longer be considered as a passive appendage to the transmission network. On the contrary, the impact of microsources on power balance and grid frequency may become much more significant over the years.
Therefore, a control and management architecture is required in order to facilitate full integration of microgeneration and active load management into the system. One promising way to realize the emerging potential of microgeneration is to take a systematic approach that views generation and associated loads as a subsystem or a microgrid.
In a typical microgrid setting, the control and management system is expected to bring about a variety of potential benefits at all voltage levels of the distribution network. In order to achieve this goal, different hierarchical control strategies need to be adopted at different network levels.
The possibility of managing several microgrids, DG units directly connected to the MV network and MV controllable loads introduces the concept of multi-microgrids. The hierarchical control structure of such a system calls for an intermediate control level, which will optimize the multi-microgrid system operation, assuming an operation under a real market environment. The concept of multi-microgrids is further developed in Chapter 5.
The potential impact of such a system on the distribution network may lead to different regulatory approaches and remuneration schemes, that could create incentive mechanisms for distribution system operators (DSOs), microgeneration owners and loads to adopt the multi-microgrid concept. This is further discussed in Chapter 7.
1.3 Clarification of the Microgrid Concept
1.3.1 What is a Microgrid?
In scope of this book, the definition from the EU research projects [7,8] is used:
Microgrids comprise LV distribution systems with distributed energy resources (DER) (microturbines, fuel cells, PV, etc.) together with storage devices (flywheels, energy capacitors and batteries) and flexible loads. Such systems can be operated in a non-autonomous way, if interconnected to the grid, or in an autonomous way, if disconnected from the main grid. The operation of microsources in the network can provide distinct benefits to the overall system performance, if managed and coordinated efficiently.
There are three major messages delivered from this definition:
- In the microgrid concept, there is a focus on local supply of electricity to nearby loads, thus aggregator models that disregard physical locations of generators and loads (such as virtual power plants with cross-regional setups) are not microgrids.
- A microgrid is typically located at the LV level with total installed microgeneration capacity below the MW range, although there can be exceptions: parts of the MV network can belong to a microgrid for interconnection purposes.
- The majority of future microgrids will be operated for most of the time under grid-connection – except for those built on physical islands – thus, the main benefits of the microgrid concept will arise from grid-connected (i.e. “normal”) operating states.
- In order to achieve long-term islanded operation, a microgrid has to satisfy high requirements on storage size and capacity ratings of microgenerators to continuous supply of all loads or it has to rely on significant demand flexibility. In the latter case, reliability benefits can be quantified from partial islanding of important loads.
- A microgrid operator is more than an aggregator of small generators, or a network service provider, or a load controller, or an emission regulator – it performs all these functionalities and serves multiple economic, technical and environmental aims.
- One major advantage of the microgrid concept over other “smart” solutions lies in its capability of handling conflicting interests of different stakeholders, so as to arrive at a globally optimal operation decision for all players involved.
A microgrid appears at a large variety of scales: it can be defined at the level of a LV grid, a LV feeder or a LV house – examples are given in Figure 1.1. As a microgrid grows in scale, it will likely be equipped with more balancing capacities and feature better controllability to reduce the intermittencies of load and RES. In general, the maximum capacity of a microgrid (in terms of peak load demand) is limited to few MW (at least at the European scale, other regions may have different upper limits, see Chapter 6). At higher voltage levels, multi-microgrid concepts are applied, implying the coordination of interconnected, but separate microgrids in collaboration with upstream connected DGs and MV network controls. The operation of multi-microgrids is discussed in Chapter 5.
1.3.2 What is Not a Microgrid?
In Figure 1.2, the microgrid concept is further clarified by examples that highlight three essential microgrid features: local load, local microsources and intelligent control. In many countries environmental protection is promoted by the provision of carbon credits by the use of RES and CHP technologies; this should be also added as a microgrids feature. Absence of one or more features would be better described by DG interconnection cases or DSI cases.
Figure 1.2 What is not a microgrid? Sample cases
In the following section, some typical misconceptions regarding microgrids are clarified:
- Microgrids are exclusively isolated (island) systems.
- Customers who own microsources build a microgrid.
- Microgrids are composed of intermittent renewable energy resources, so they must be unreliable and easily subject to failures and total black-outs.
- Microgrids are expensive to build, so the concept will be limited to field tests or only to remote locations.
- The microgrid concept is just another energy retailer advertising scheme to increase his income.
- The microgrid controllers will force consumers to shift their demand, depending on the availability of renewable generation, e.g. to switch on the washing machine at home only when the sun is shining or the wind is blowing.
- A microgrid is such a totally new idea, that system operators need to rebuild their entire network.
- Microgrid loads will never face any supply interruptions.
1.3.3 Microgrids versus Virtual Power Plants
A virtual power plant (VPP) is a cluster of DERs which is collectively operated by a central control entity. A VPP can replace a conventional power plant, while providing higher efficiency and more flexibility. Although the microgrid and the VPP appear to be similar concepts, there are a number of distinct differences:
- Locality – In a microgrid, DERs are located within the same local distribution network and they aim to satisfy primarily local demand. In a VPP, DERs are not necessarily located on the same local network and they are coordinated over a wide geographical area. The VPP aggregated production participates in traditional trading in normal energy markets.
- Size – The installed capacity of microgrids is typically relatively small (from few kW to several MW), while a VPP's power rating can be much larger.
- Consumer interest – A microgrid focuses on the satisfaction of local consumption, while a VPP deals with consumption only as a flexible resource that participates in the aggregate power trading via DSI remuneration.
Following on from the definition of a VPP as a commercial entity that aggregates different generation, storage or flexible loads, regardless of their locations, the technical VPP (TVPP) has been proposed, which also takes into account local network constraints. In any case, VPPs, as virtual generators, tend to ignore local consumption, except for DSI, while microgrids acknowledge local power consumption and give end consumers the choice of purchasing local generation or generation from the upstream energy market. This leads to a better controllability of microgrids, as shown in Figure 1.3, where both supply and demand resources of a microgrid can be simultaneously optimized, leading to better DG profitability.
Figure 1.3 Microgrid benefit over commercial and technical VPP due to supply side integration
1.4 Operation and Control of Microgrids
1.4.1 Overview of Controllable Elements in a Microgrid
As well as the basic demand and supply resources, a microgrid can potentially be equipped with energy balancing facilities, such as dispatchable loads (e.g. electric vehicles) and substation storage units (Figure 1.4), that could either contribute to minimization of power exchange or maximization of trading profit (in case of free exchange under favorable pricing conditions).
Figure 1.4 Microgrid stakeholders
1.4.1.1 Intermittent RES Units
Controllability of intermittent RES units is limited by the physical nature of the primary energy source. Moreover, limiting RES production is clearly undesirable due to the high investment and low operating costs of these units and their environmental benefits over carbon emission. Consequently, it is generally not advisable to curtail intermittent RES units, unless they cause line overloads or overvoltage problems.
The operation strategy for intermittent RES units can therefore be described as “priority dispatch”, that is, intermittent RES units are generally excluded from the unit commitment schedule, as long as they do not violate system constraints. Units with independent reactive power interfaces (decoupled from the active power output) can be included in reactive power dispatch to improve the technical performance of the total microgrid.
1.4.1.2 Dispatchable Microsource and CHP Units
Controllability of CHP units varies according to the way they meet local heat demand – that is, they can be heat-driven, electricity-driven or operated in a hybrid mode. Since most microsource and CHP units are based on rotating machine technology, their reactive power output will be constrained by both the active power output and the apparent power rating.
Due to improved controllability of dispatchable microsource units, a microgrid with multiple microsource units will need to solve the traditional unit commitment problem – albeit at a much smaller scale. On the other hand, the microgrid operator needs to cope with much higher net load variations (i.e. load minus intermittent RES output). At the same time, optimization constraints will likely include grid operating states and emission targets, which add much more complexities to the unit commitment task.
1.4.1.3 Storage Units
Technically, a storage unit could behave either under a load-following paradigm (i.e. balancing applications) or under a price-following paradigm (i.e. arbitrager applications) depending on the purpose of its operation. At the same time, storage units can provide balancing reserves ranging from short-term (milliseconds to minute-level) to long-term (hourly to daily scale) applications. Specifically, for DC-based storage technologies (battery, super-capacitor etc.), a properly designed power electronic interface could contribute to the reactive power balance of the system without incurring significant operational costs.
1.4.1.4 Demand Side Integration
Demand side integration is also referred to as demand side management (DSM) or demand side response (DSR). It is based on the concept [13] that customers are able to choose from a range of products that suit their preferences. The innovative products packaged by energy suppliers will deliver – provided that end-user price regulation is removed – powerful messages to consumers about the value of shifting their electricity consumption. Examples of such offers include
- time-of-use (ToU): higher “on-peak” prices during daytime hours and lower “off-peak” prices during the night and at weekends (already offered in some EU member states)
- dynamic pricing (including real-time pricing): prices fluctuate to reflect changes in the wholesale prices
- critical peak prices: same rate structure as for ToU, but with much higher prices when wholesale electricity prices are high or system reliability is compromised.
The control of customers load can either be
- manual: customers are informed about prices, for example on a display, and decide on their own to shift their consumption, perhaps remotely through a mobile phone
- automated: customers' consumption is shifted automatically through automated appliances, which can be pre-programmed and can be activated by either technical or price signals (as agreed for instance in the supply contract).
DSI measures in a microgrid are based on forecasts of load and RES outputs and will very probably vary from day to day. A requirement for the successful application of microgrid DSI measures is the adoption of smart metering and smart control of household, commercial and agricultural loads within the microgrid. Depending on the criticality of the target load, DSI measures can generally be divided into shiftable loads and interruptible loads. The integration of DSI measures is expected to maximize their benefits in potential “smart homes”, “smart offices” and “smart farms” within microgrids.
1.4.2 Operation Strategies of Microgrids
Currently available DG technologies provide a wide variety of different active and reactive power generation options. The final configuration and operation schemes of a microgrid depend on potentially conflicting interests among different stakeholders involved in electricity supply, such as system/network operators, DG owners, DG operators, energy suppliers, customers and regulatory bodies. Therefore, optimal operation scheduling in microgrids can have economic, technical and environmental objectives (Figure 1.5).
Figure 1.5 Microgrid operation strategies
Depending on the stakeholders involved in the planning or operation process, four different microgrid operational objectives can be identified: economic option, technical option, environmental option and combined objective option.
In the economic option, the objective function is to minimize total costs regardless of network impact/performance. This option may be envisaged by DG owners or operators. DGs are operated without concern for grid or emission obligations. The main limitations come from the physical constraints of DG.
The technical option optimizes network operation (minimizing power losses, voltage variation and device loading), without consideration of DG production costs and revenues. This option might be preferred by system operators.
The environmental option dispatches DG units with lower specific emission levels with higher priority, disregarding financial or technical aspects. This is preferred for meeting environmental targets, currently mainly supported by regulatory schemes. DG dispatch is solely determined by emission quota; only DG physical limitations are considered.
The combined objective option solves a multi-objective DG optimal dispatch problem, taking into account all economic, technical and environmental factors. It converts technical and environmental criteria into economic equivalents, considering constraints from both network and DG physical limits. This approach could be relevant, for instance, to actors that participate not only in classical energy markets, but also in other potential markets for provision of network services and emission certificates.
1.5 Market Models for Microgrids
1.5.1 Introduction
Microgrids are required to function within energy markets. Current energy markets operate with various levels of complexity ranging from fully regulated to fully liberalized models. A related issue is the interdependency between competitive (generation, retail) and regulated activities (transmission and distribution) in market structures that range from vertically integrated utilities to full ownership unbundling of these activities, as implemented currently in Europe. Therefore, definition of the various actors participating in the energy market, especially at the distribution level, are by far not generally agreed. Within this context, it is very difficult to define a “generic” energy market model. The following discussion provides a general overview of possible market models for microgrids.
Depending on the operational model, two major markets can be distinguished: the wholesale market and the retail market. These two different markets can function interacting with each other via a pool or/and via bilateral transactions. Traditionally, mandatory open transmission access to generators and energy importers has created more competitive wholesale power markets. At the retail level, competition has been established in many countries, which gives customers additional choices in the supply and pricing of electricity. Due to their relatively small sizes, microgrids cannot participate directly in the wholesale or retail market, thus they can possibly enter as part of a portfolio of a retail supplier or an energy service company (ESCO). Direct control of DERs by DSOs is another possible model. Fair competition in the retail sector is expected to serve as the basis of microgrid adoption, which assumes a suitable regulatory environment and an ICT infrastructure capable of supporting the market operation (e.g. electronic/smart meters and a standardized interaction between retail companies and DSOs), as further discussed in Chapter 2. These are essential conditions for the successful implementation of microgrids.
The relevant key actors in the energy market are as follows:
- Consumer: The consumer can represent a household or a medium or small enterprise. Typically the consumer has a contract with a retail company for his energy supply. Furthermore, the consumers, or anyone connected to the distribution network, need to pay fees to the distribution network owner for using the network.
- DG owner/operator: Typically the owners of the DG units are also responsible for their operation. DG owners inject their production to the network, possibly enjoying priority dispatch and fixed feed-in tariffs, especially for RES-based DG or they might have contracts with a retail company. They may pay distribution network charges. It is assumed that some or all of the DG units will be equipped with at least some monitoring and possibly control capabilities.
- Prosumer: This is the special case of consumers who have installed small DG in their premises. The local DG production can cover, wholly or partly, the consumption of the owner, and the surplus can be exported to the main power grid. Alternatively, the total local production can also be sold directly to the main power grid enjoying favorable feed-in tariffs.
- Customer: Consumers, DG owners/operators and prosumers are termed customers.
- Market regulator: A regulatory authority for energy is an independent body responsible for the open, fair and transparent operation of the market, ensuring open access to the network and efficient allocation of network costs. Depending on local conditions, it also approves the level of network usage charges and in some cases end-user prices.
- Retail supplier, energy service company (ESCO): A supplier directly interacts with the customers and has contracts with them. Its main duties are to provide electricity and possibly other energy services to its customers. Regarding electricity retail activities, the supplier acquires energy from different sources, such as the wholesale market or spot market or local DG production. Unless partly or wholly regulated, the supplier determines the energy prices of the electricity delivered to the customer, which may vary depending on time and location. In the context of microgrids, suppliers are the actors to maximize the value of the aggregated DER participation in local energy markets, thus maximizing the microgrid's value. Suppliers are also responsible for translating the complexity and sophistication of the retail market, including the demand response market and DER remuneration schemes, into simple forms that customers demand, by “packaging” attractive products. The products offered should be easy for the customer to understand and suppliers should effectively manage any complexity in costs (e.g. variable grid tariffs). Depending on the regulatory framework and the market conditions, suppliers might offer, next to the electricity supply, a broader range of energy services featuring better reliability, appealing (green) energy production and convenience. In the future, microgrids can be part of an ESCO commercial business portfolio, which includes a broad range of comprehensive energy solutions, aiming to reduce the holistic energy cost of a group of buildings or a building complex. Depending on the business case, the supplier/ESCO might need to install the necessary home gateway that will be responsible for appliance management.
- Distribution system operator (DSO): The distribution system operator is the actor responsible for the operation, maintenance and development of the distribution network in a given area. Usually the DSO (a) manages the HV, MV and LV distribution systems, (b) is obliged to deliver electricity to consumers or absorb energy from DG/RES and (c) being a regulated entity, it is not involved in any retail activity. In a future power system characterized by smart grids and load flexibility, DSOs will provide the playing field where suppliers can offer innovative products, and they will therefore play the role of neutral market facilitators. Where applicable, the role of DSOs as metering operators is crucial for the efficient delivery of energy services offered by suppliers on a competitive basis. Suppliers will need timely, transparent and non-discriminatory access to commercial data, while DSOs have access to the technical data necessary to manage their grid effectively. Taking into account that microgrids provide considerable flexibility, DSOs will contract both with suppliers managing microgrid customers and with individual distributed generators to utilize their flexibility for local network balancing. Where applicable, network tariffs should support microgrids' commercial participation and more generally, the development of demand response products. The direct control of demand response or DER (e.g. storage, installed at the distribution level) without involving suppliers is another possibility, although it would threaten the suppliers' ability to balance their portfolios, increasing the need for contracting reserves, and this in turn would lead to increased costs for consumers. In the future, it is anticipated that a new set of agreements between suppliers and DSOs will ensure that customers benefit from proper functioning of the market, smooth processes and a secure and reliable electricity supply; suppliers will market new products and optimize their supply and balancing portfolio, while DSOs can guarantee local grid stability and security of supply through system services.
- Microgrid operator: The microgrid operator is responsible for the operation, maintenance and development of the local (LV) distribution network forming the microgrid. Depending on the business model, discussed in Section 1.4.2, this role can be assumed by the local DSO or it can be a dedicated, independent DSO who acts locally on behalf of the microgrid customers.
Microgrids' participation in energy markets can be analyzed from the generation and the demand side.
1.5.1.1 DG/RES Generation
As discussed in the previous section, the production of DG sources within a microgrid can be dispatchable or intermittent for certain RES technologies, such as PVs and small wind turbines. Today, in most countries, there is no requirement to control the production of RES units at the distribution level. The DSO is obliged to absorb all the energy produced, except for security reasons, and most RES technologies enjoy favorable feed-in-tariffs for their production. This framework provides no incentives to the DG owners for active management of their units or for actually integrating them in microgrid operation.
1.5.1.2 Demand Side
Demand side integration is an important feature of microgrid operation, as discussed in Section 1.4.1. Demand response can be classified according to the way load changes are induced [14]:
- Price-based demand response implementations induce changes in usage by customers in response to time-based changes in the prices they pay. This includes real-time pricing, critical-peak pricing and time-of-use rates.
- Incentive -based demand response programs are facilitated by utilities, retail companies or a regional DSO. These programs give customers load reduction incentives that are separate from, or in addition to, their retail electricity rates, regardless of whether the rates are fixed (based on average costs) or time-varying. Load reductions are coordinated as requested by the program operator and can include direct load control, that is, a program through which the program operator remotely shuts down or cycles a customer's electrical equipment (e.g. air conditioner, water heater, space heating) at short notice.
The goal of both policies is to smooth the daily load curve of the system by shifting load. This includes peak shaving (limit consumption during hours of peak load) and valley filling (increase consumption during the hours of low demand). Load shifting can be also used to compensate the volatility in RES production or in the electricity prices on the spot market. In general, flexible demand is an obvious source of “negative” power reserves, offering a great potential that has not so far been adequately explored in practice.
1.5.1.3 Ancillary Services
“Ancillary services are all services required by the transmission or distribution system operator to enable them to maintain the integrity and stability of the transmission or distribution system as well as the power quality” [15]. The services include both mandatory services and services subject to competition. The increased penetration of DER in the distribution network combined with the objective of more reliable and cost-efficient network operation, have opened up new opportunities for meeting system obligations and providing grid services (reactive power, voltage support, congestion management) which are required by DSOs at the distribution level. Ancillary services are classified according to the operation modes of microgrids (normal operation or emergency operation/grid-connected operation or islanded operation), as follows:
- grid-connected operation:
- islanded operation:


The participation of microgrids in local ancillary service markets can be seen as a great opportunity, especially regarding active reserves and voltage support. DER capabilities and detailed technical solutions for the provision of ancillary services are discussed in the following chapters of this book, but the necessary regulatory environment needs to be applied, and a number of critical questions need to be answered, such as:
- What is the cost of provision of ancillary services?
- Who is responsible for coordinating the associated functions?
1.5.2 Internal Markets and Business Models for Microgrids
The internal market of a microgrid mainly relates to the ownership and business models established between major stakeholders involved in the operation of a microgrid, such as local consumers, microsources, DSO and energy supplier. A microgrid's internal market mainly determines the amount and direction of cash flows within the microgrid. It also defines which entity will participate in external markets, as representative of the whole group of stakeholders. The structure of an internal market, however, does not necessarily impact the choice of microgrid operation strategy or its collective behavior, as seen from the external grid; thus two microgrids with completely different ownership properties might behave very similarly to the external energy market.
Microgrid structures can be differentiated by the level of their DER aggregation: they range from a simple collection of independent market players to a collaboration that encompasses all demand- and supply-side entities. Similar to the unbundling of central generation from transmission network, the operation structure of a microgrid will be mainly decided by the ownership of microsources, that is, by the DSO, the end consumer, the energy supplier or directly by the microsource operator as independent power producer (IPP) (Figure 1.6).
Figure 1.6 Sample micro-source ownership possibilities in a microgrid
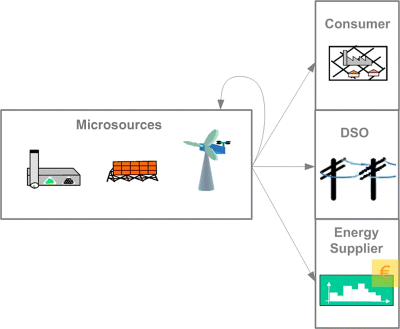
Although theoretically there could be numerous forms of microgrids, three typical setups can be identified, named as the DSO monopoly, free market and prosumer consortium models. These are discussed in the following subsections. In all models it is assumed that all the required metering, monitoring and control functionalities are provided.
1.5.2.1 DSO Monopoly Model
In a DSO monopoly microgrid, the DSO is part of a vertically integrated utility, so it not only owns and operates the distribution grid, but also fulfills the retailer function of selling electricity to end consumers. Within this single-player context, integration and operation of microsources is most conveniently also undertaken by the DSO, which leaves almost all technical and financial consequences (i.e. both costs and benefits) of microgrid conversion to DSO responsibility (Figure 1.7). In unbundled systems, DSOs might not be allowed to own and operate a DER, but a DER used exclusively for supporting distribution system operation might be an exception.
Figure 1.7 DSO monopoly model
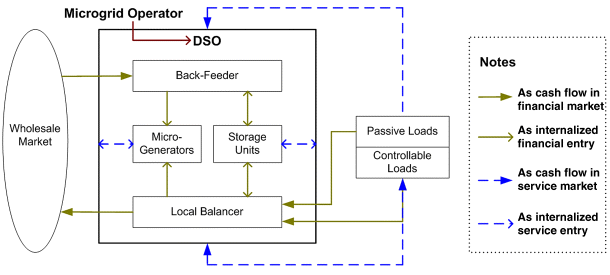
In a DSO monopoly microgrid, microgeneration units tend to be larger, and storage units tend to be located at central substations.
With the business model of Figure 1.7, a versatile “extended” DSO plays the role of both physical and financial bridge between the overlying grid and end consumers. Microsource control decisions are basically made within the framework of DSO functionalities. There is no room for a local service market except for tariff-driven DSI programs.
In general, a DSO monopoly microgrid is most likely to be built upon a technically challenged distribution grid with aging infrastructure, maintenance and/or supply quality problems. The investment decision in microsource units by a DSO (if allowed by the market regulator) can be generally explained as an alternative to more expensive network solutions, such as upgrading overloaded lines to overcome thermal constraints. Therefore, the potential profitability of selling microsource energy to local consumers may not be the primary consideration.
1.5.2.2 Liberalized Market Model
In liberalized markets, microgrids (Figure 1.8) can be driven by various motives (economic, technical, environmental etc.) from various stakeholders (suppliers, DSO, consumers etc.). As discussed in Section 1.5.1, suppliers/ESCOs are the actors best suited to maximize the value of the aggregated DER participation in local liberalized energy markets, that is, for the commercial participation of microgrids. The daily operational decisions depend on real-time negotiations (i.e. interest arbitration) of all parties involved. In this case, a microgrid operator or central controller (MGCC) is responsible for microgrid local balance, import and export control, technical performance maintenance and emission level monitoring. In a free market microgrid, DG and storage units can vary in forms, sizes and locations.
Figure 1.8 Free market model
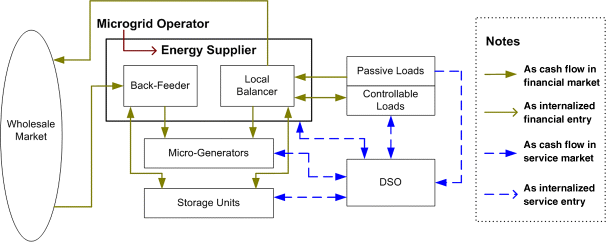
1.5.2.3 Prosumer Consortium Model
A prosumer consortium microgrid (Figure 1.9) is most likely to be found in regions with high retail electricity price and/or high microgeneration financial support levels. In this case, single or multiple consumers own and operate microsources to minimize electricity bills or maximize sales revenue from energy export to the upstream network. This type of microgrid tends by nature to minimize the use of the distribution grid (which leads to a reduction in the use of system revenues) and may neglect network constraints (i.e. DG hosting capacity) during its design. DSOs can only passively influence the operation of a prosumer consortium microgrid by imposing requirements and charges on the microsource owners, but will not be able to benefit from the local trading process.
Figure 1.9 Prosumer consortium model
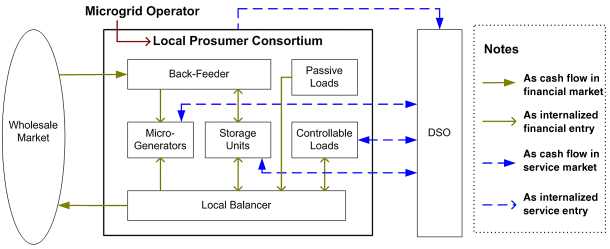
In a prosumer consortium microgrid, microgeneration tends to be smaller, and storage tends to be small and dispersed (e.g. plug-in electric vehicles).
1.5.2.4 Costs and Revenues for a Microgrid Owner
Despite individual differences, for all three examined ownership models a common objective function can be defined as
minimize: target value = self supply cost − export profit (if applicable)
- self supply cost = import energy cost + microsource or local DER production cost for self supply
- export profit = export sales revenue − microsource or local DER production cost for back-fed energy
This target value can be understood as the sum of opportunity costs minus opportunity revenues obtained from optimal real-time dispatch decisions between internal (microgrid) and external (market) resources. Of course, for each microgrid ownership model examined, extra internal cost entries will arise due to the differences of corresponding interest allocation models.
1.5.3 External Market and Regulatory Settings for Microgrids
One critical factor of the financial and technical feasibility of microgrids is external market operation and the associated regulatory rules. As all investment decisions are de facto based on expected profitability, the external environment of a microgrid has a major impact over whether or not a commercial-level microgrid will be realized. Public policy support for microgrids in order to promote technological evolution will also prove to be critical for creating a level playing field for both existing and new players in the market.
As briefly introduced in the previous section, there are two main types of external markets with impact on a microgrid: the energy market and the ancillary services market. It is clear that the ancillary services market can be an ideal playing field for microgrids owing to their controllability, when properly designed. In the following part of this section focus is placed on the three main factors of external energy market operation that have critical influence on a microgrid:
1.5.3.1 Satisfaction of Local Consumption
Satisfaction of local consumption implies that the power produced by microgeneration is used to supply partly or wholly in-site consumption. In such a case, there is no requirement for separate metering of microsource generation (also called “net-metering”). However, on-site generation and on-site load need to be metered separately when microsource units appear as independent generators that sell all their production directly to the network and are not financially related to end consumers. In this case, local consumption is a market opportunity that can be easily overlooked by all players (Figure 1.10).
Figure 1.10 Impact of local balancing
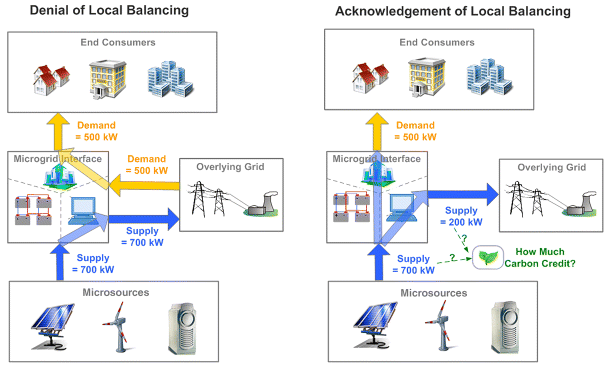
There are two main advantages of promoting local consumption satisfaction within a microgrid:
The local retail market concept is therefore directly linked to the local consumption mechanism, which can also be seen as a two-sided hedging tool for both demand and supply players for reducing market risk: consumers can use the local market to hedge against high market price, while microsources can use the local market to hedge against low market price.
1.5.3.2 Recognition of Locational Value
The microgrid operator or retail company that undertakes the microgrid market participation is likely to trade with the external market on a daily basis, which implies both purchase and selling of electricity. Under this general setting, a microgrid is likely to be faced with wholesale prices for export and (quasi) retail prices for import (directional pricing), unless a different regulatory framework is in place.
In the microgrid context, the difference between purchase and selling prices is attributed to the network charges or use of system (UoS) charges, which is applied on top of the electricity prices in the wholesale market. However, because microsources are located closer to the consumption, they do not require the same transmission and distribution network infrastructures. The locational value of the microsources could be recognized by allowing a total or partial exemption of UoS charge that can eventually improve the profitability of these microsources against the more competitive large, central units. In fact, the exemption from UoS charges can be seen as a security of supply compensation to be paid by the DSOs, in order to avoid or defer investments for expensive network upgrades.
Depending on the level of political and social support for microgrids, DSOs may also agree to lower UoS charges imposed on power trades between microgrids and external generators or loads – especially when the power is exchanged between one microgrid and another, or with a DG or controllable load at the MV level. The basic reasoning behind this is to extend the internal production–consumption balance of a microgrid to multi-microgrids – the aggregation of microgrids in conjunction with an MV level DG and controllable loads that are comparable in size with a complete LV microgrid. In this way (shown in Figure 1.11), selling and buying prices both within and outside of a microgrid can be unified to promote bidirectional trading on a real-time basis (uniform pricing).
Figure 1.11 Energy pricing schemes for microgrids
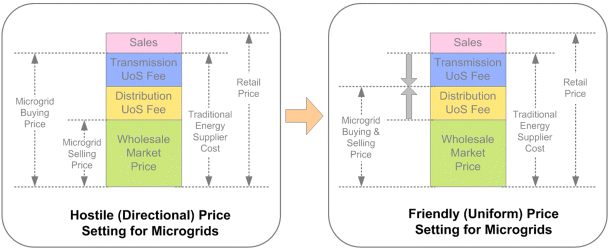
1.5.3.3 Fixed versus Time of Use Tariffs
Electricity pricing can be fixed, that is, a microgrid is subject to a fixed selling price and a fixed buying price (under a uniform pricing scheme both prices coincide) for participation in the energy market or varying with time –time of use (ToU) prices – that is, a microgrid obtains hourly (or time-of-day block) prices for both selling and buying electricity. It is clear that the adoption of ToU prices offers greater flexibility and favors local resources and thus the adoption of microgrids.
1.5.3.4 Example
The following practical example demonstrates in a simple way the importance of the above factors on the profitability of microgrids. By combining the satisfaction of local consumption, the recognition of locational value and the adoption of ToU tariff criteria, eight potential external market environments can be derived. Out of them one reference case and three main microgrid-enabling cases are shown in Table 1.1.
Table 1.1 Microgrid pricing schemes.
The reference case refers to a distribution network where local DG production is ignored and local consumption is supplied by energy imported at fixed retail prices. In the (forced) island case, the local DG units have economic incentive to feed local consumption at their production costs, but are discouraged from exporting due to low remuneration tariff. In the hybrid case, energy from the external market, if cheaper, is bought at real-time retail price, while in the exchange case the consumption is supplied by the optimal combination of energies bought from the internal and external market at “uniform” prices, assumed to be the average between wholesale and retail prices. For both hybrid and exchange cases, power export becomes a feasible option due to the potential price peaks. In order to illustrate potential economic consequences of the four proposed pricing scenarios, a four-hour, two-unit dispatch problem is analyzed with the following assumptions: mean wholesale price is assumed to be 7.5 Ct/kWh, mean retail price is 13.5 Ct/kWh. Unit 1 is rated at 30 kW with 12 Ct/kWh, unit 2 is rated at 15 kW with 15 Ct/kWh. Hourly demands are assumed to be 15 kWh, 35 kWh, 25 kWh and 5 kWh from hours 1 to 4.
In Figure 1.12, the per kWh electricity costs in the sample microgrid are compared for the four scenarios. It is shown that the cost decreases as price flexibility and transparency improves. The exchange case is proven to provide the best financial conditions for a microgrid, as it provides the lower cost for electricity supply within the system. The (forced) island case provides the worst financial conditions for the formation of the microgrid, while in the hybrid case the cost lies between the island and exchange cases following variations of real-time prices.
Figure 1.12 Per kWh electricity costs under different pricing strategies

This example suggests that a large installation of microsource capacity that exceeds peak load demand could provide export potential of excess generation. If the microsource units in a microgrid are too small to achieve reversal of power flow, the eventual cost difference between hybrid and exchange case will be significantly reduced and might even lead to lower costs of the hybrid case compared to the exchange case.
1.6 Status Quo and Outlook of Microgrid Applications
Currently, an increasing number of microgrid pilot sites can be observed in many parts of the world, as described in Chapter 6 of this book. It is true, however, that up to now, cost, policy and technology barriers have largely restrained the wide deployment of microgrids in distribution networks owing to their limited commercial appeal or social recognition. However, these three barriers are currently undergoing considerable changes – they are very likely to turn into key enablers in the future, eventually leading to a widespread microgrid adoption worldwide.
Firstly, the cost factor might prove to be the most effective driving force for microgrids in the very near future. This might happen not only because of the reduction of microsource costs, but also because of the relative changes of external opportunity costs due to economic (fluctuating market prices), technical (aging of network infrastructure) and environmental (emission trading) factors.
When microsource penetration at a LV grid becomes significant, participants in the electricity retail business will consider the aggregated power from small generators as a new market opportunity. Unlike in the case of VPP, microgrid stakeholders will eventually recognize a unique feature of aggregated microsource units, namely locality: the microsource units can potentially sell directly to end consumers in an “over-the-grid” manner. In order to turn this potential into reality, however, the second factor – appropriate policy and regulatory environment – is needed to enable the operation of a local market within a microgrid.
Finally, the adoption of favorable selling prices in local retail markets will attract even more microsource units, allowing the microgrid to operate islanded, if beneficial. With the help of smart metering, control and communication technologies, the microgrid operator will eventually be able to coordinate a large consortium of intermittent and controllable microsource units, as well as central and distributed storage devices, to achieve multiple objectives and, at the same time, to cater for the interests of different stakeholders. Figure 1.13 provides an exemplary roadmap for microgrid development in Europe [8].
Figure 1.13 Roadmap for microgrid development
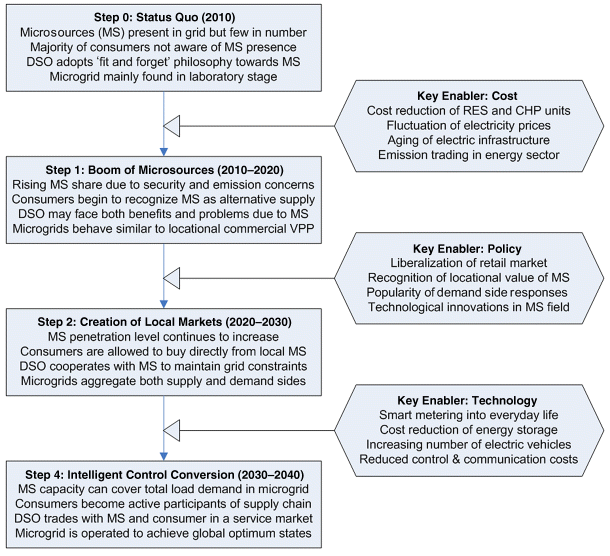
1. CIGRE (June 2011) Report on behalf of the Technical Committee. “Network of the Future”, Electricity Supply Systems of the Future, Electra, No 256.
2. Smartgrids, European Technology Platform on Vision and Strategy for Europe's Electricity Networks of the Future. s.l.: EUR 22040, 2006.
3. Peças Lopes, J.A., Hatziargyriou, N., Mutale, J.et al. (2007) Integrated distributed generation into electric power systems: A review of drivers, challenges and opportunities. Elsevier Electr. Pow. Syst. Res., 77 (9).
4. Pepermans, G., Driesen, J., Haeseldonckx, D.et al. (2005) Distributed generation: definition, benefits and issues. Int. J. Energy Policy, 33 (6), 787–798.
5. Lasseter, R., Akhil, A., Marnay, C.et al. (April 2002) White paper on Integration of Distributed Energy Resources – The CERTS MicroGrid Concept. s.l.: Office of Power Technologies of the US Department of Energy, Contract DE-AC03-76SF00098.
6. Lasseter, R.H. (2002) MicroGrids. IEEE Power Engineering Society Winter Meeting Conference Proceedings, vol. 1., New York, NY: s.n., pp. 305–308.
7. “Microgrids: Large Scale Integration of Micro-Generation to Low Voltage Grids”, ENK5-CT-2002-00610. 2003–2005.
8. “More microgrids: Advanced Architectures and Control Concepts for More microgrids”, FP6 STREP, Proposal/Contract no.: PL019864. 2006–2009.
9. Hatziargyriou, N. (2004) MicroGrids. 1st International Conference on the Integration of Renewable Energy Sources and Distributed Energy Resources (IRED). Brussels: s.n., 1–3 December.
10. Hatziargyriou, N., Asano, H., Iravani, R. and Marnay, C. (July/August 2007) microgrids: An Overview of Ongoing Research, Development and Demonstration Projects. IEEE Power and Energy, Vol. 5, Nr. 4, pp. 78–94.
11. Hatziargyriou, N. (May–June 2008,) Microgrids [guest editorial]. IEEE Power and Energy Magazine, Volume 6, Issue 3, pp. 26–29.
12. Karfopoulos, E.L.et al. (25–28 Sept 2011) Introducing electric vehicles in the microgrids concept. 16th International Conference on Intelligent Systems Application to Power Systems, Hersonissos, Heraklion, Greece.
13. Eurelectric Policy Paper. “Customer-Centric Retail Markets: A Future-Proof Market Design ”. September 2011.
14. CIGRE WG C6.09. “Demand Side Integration ”, Technical Brochure, August 2010.
15. Eurelectric, Thermal Working Group (February 2004) Ancillary Services: Unbundling Electricity Products – an Emerging Market, Ref: 2003-150-0007.