A.3. FORTRAN Program for Mann–Kendal Test
$DEBUG
C PRGRAMME FOR IDENTIFICATION OF TREND USIN MANN KENDALLS TEST
C WHEN SERIES IS AUTOCORRELETED. IN THIS APPROACH THE TIME SERIES
C IS NORMALIZED USING THE LAG-1 AUTO CORRELATION FUNCTION OF TIME SERIES
DIMENSION X(100,1000),TITLE (80),x1(1000),Y1(1000)
DIMENSION R(50),RKL(50),RKU(50)
OPEN(UNIT=1,FILE=‘Rainfall.IN’,STATUS=‘OLD’)
OPEN(UNIT=2,FILE=‘Surwania_Rainfall_MK_ACF.OUT’)
OPEN(UNIT=3,FILE=‘Surwania_Rainfall_MK_ACF_SUMMARY.OUT’)
READ(1,1)TITLE
1 FORMAT(80A1)
C N IS TOTAL NO. OF DATA POINTS IN THE SERIES.
read(1,∗)m,n
c READ(1,∗)N
C X IS THE INPUT SERIES.
do j=1,n
READ(1,∗)(X(I,j),i=1,m)
enddo
C
do j=1,n
WRITE(2,2)(X(I,j),i=1,m)
enddo
C
2 FORMAT(1X,(2X,10F7.1))
WRITE(∗,∗)‘SUMMARY OF TREND ANALYSIS USING MANN KENDALL TEST’
WRITE(∗,∗)‘=========================================’
WRITE(∗,∗)
WRITE(∗,∗)‘NO. OF TIME SERIES =’,M
WRITE(∗,∗)‘NO. OF OBSERVATIONS =’,N
WRITE(∗,∗)
WRITE(∗,∗)‘---------------------------------’
WRITE(∗,∗)‘I RKL(1) R(1) RKU(1) ICODE Z COMMENT’
WRITE(∗,∗)‘---------------------------------’
WRITE(3,∗)‘SUMMARY OF TREND ANALYSIS USING MANN KENDALL TEST’
WRITE(3,∗)‘=========================================’
WRITE(3,∗)
WRITE(3,∗)‘NO. OF TIME SERIES =’,M
WRITE(3,∗)‘NO. OF OBSERVATIONS =’,N
WRITE(3,∗)
WRITE(3,∗)‘---------------------------------’
WRITE(3,∗)‘I RKL(1) R(1) RKU(1) ICODE Z COMMENT’
WRITE(3,∗)‘---------------------------------’
do i=1,m
WRITE(2,∗)‘∗∗∗∗∗∗∗∗∗∗∗∗∗∗∗∗∗∗∗∗∗∗∗∗∗∗∗∗∗∗∗∗∗∗∗∗∗∗∗∗∗∗∗∗∗∗∗’
WRITE(2,∗)‘CLIMATIC SERIES=’,I
WRITE(2,∗)‘∗∗∗∗∗∗∗∗∗∗∗∗∗∗∗∗∗∗∗∗∗∗∗∗∗∗∗∗∗∗∗∗∗∗∗∗∗∗∗∗∗∗∗∗∗∗’
do j=1,n
x1(j)=x(i,j)
enddo
CALL ACF(N,X1,R,RKL,RKU)
DO J=1,N-1
Y1(J)=X1(J+1)-R(1)∗X1(J)
ENDDO
IF (R(1).LT.RKL(1).OR.R(1).GT.RKU(1))THEN
ICODE=2 !R1 IS SIGNIFICANT
call mann kendal(n,Y1,ns,vars,z,np,nl,ntie)
ICODE_T=‘NO’
ELSE
ICODE_T=‘YES’
ENDIF
WRITE(∗,4)I,RKL(1),R(1),RKU(1),ICODE,Z,ICODE_T
WRITE(3,4)I,RKL(1),R(1),RKU(1),ICODE,Z,ICODE_T
ELSE
ICODE=1 !R1 IS NOT SIGNIFICANT
CALL MANN KENDAL(N,X1,NS,VARS,Z,NP,NL,NTIE)
IF(ABS(Z).LE.1.96)THEN
ICODE_T=‘NO’
ELSE
ICODE_T=‘YES’
ENDIF
WRITE(∗,4)I,RKL(1),R(1),RKU(1),ICODE,Z,ICODE_T
WRITE(3,4)I,RKL(1),R(1),RKU(1),ICODE,Z,ICODE_T
ENDIF
ENDDO
4 FORMAT(1X,I3,3X,F5.3,2X,F5.3,2X,F5.3,6X,I1,1X,F8.3,3X,A5)
WRITE(∗,∗)‘---------------------------------’
WRITE(3,∗)‘---------------------------------’
stop
end
subroutine mann kendal(n,x,ns,vars,z,np,nl,ntie)
dimension X(1000)
NP=0
NL=0
NTIE=0
DO 51 I=1,N
J=I+1
NT=1
DO 52 I1=J,N
IF(X(I1).GT.X(I))NP=NP+1
IF(X(I1).EQ.X(I))THEN
NT=NT+1
WRITE(2,∗)‘XI=’,X(I1)
ELSE
IF(X(I1).LT.X(I))NL=NL+1
ENDIF
52 CONTINUE
NTIE=NTIE+NT∗(NT-1)∗(2∗NT+5)
51 CONTINUE
NS=(NP-NL)
VARS=(N∗(N-1)∗(2∗N+5)-NTIE)/18
IF(NS.GT.0) THEN
ELSEIF(NS.LT.0)THEN
Z=(NS+1)/SQRT(VARS)
ELSE
Z=0
ENDIF
WRITE(2,13)n,NTIE,VARS
13 FORMAT(‘no. of observations=’i4/’ VALUE OF TIE=‘I5/’
1VAR(S)=‘F15.5)
WRITE(2,3)NS,Z
3 FORMAT(‘ VALUE OF S=‘I5/’ TEST STATISTICS=’F10.5)
IF(Z.LT.-1.96)WRITE(2,4)
IF(Z.GE.1.96)WRITE(2,5)
IF(ABS(Z).LT.1.96)WRITE(2,6)
4 FORMAT(‘ THERE IS FALLING TREND IN DATA AT 5% SIGNIFICANCE 1LEVEL’)
5 FORMAT(‘ THERE IS RISING TREND IN DATA AT 5% SIGNIFICANCE 1LEVEL’)
6 FORMAT(‘ THERE IS NO TREND IN DATA AT 5% SIGNIFICANCE LEVEL’)
c write(2,7)(x(i),i=1,n)
7 format(f8.1)
return
END
C PROGRAM TO COMPUTE THE AUTO-CORRELATION FUNCTION
C PROGRAM IS BASED ON THE EQUATION GIVEN BY SALAS ET.AL.(1988)
C PROGRAM WRITTEN BY R.K.RAI
C
SUBROUTINE ACF(N,X,R,RKL,RKU)
DIMENSION X(500),Y(500),R(50),RKL(50),RKU(50)
K=N/2
C
WRITE(2,∗)‘--------------------------------’
WRITE(2,∗)‘LAG(K) LOWER LIMIT R(K) UPPER LIMIT’
WRITE(2,∗)‘--------------------------------’
DO KK=1,K
SUMX=0.0
SUMY=0.0
SUMX2=0.0
SUMY2=0.0
SUMXY=0.0
NK=N-KK
DO J=1,N-KK
Y(J)=X(J+KK)
SUMY=SUMY+Y(J)
SUMX=SUMX+X(J)
DO J=1,N-KK
SUMX2=SUMX2+(X(J)-SUMX/NK)∗(X(J)-SUMX/NK)
SUMY2=SUMY2+(Y(J)-SUMY/NK)∗(Y(J)-SUMY/NK)
SUMXY=SUMXY+((X(J)-SUMX/NK)∗(Y(J)-SUMY/NK))
ENDDO
RKL(KK)=(-1-1.645∗SQRT(N-KK-1.))/(N-KK)
R(KK)=SUMXY/(SQRT(SUMX2)∗SQRT(SUMY2))
RKU(KK)=(-1+1.645∗SQRT(N-KK-1.))/(N-KK)
WRITE(2,4)KK,RKL(KK),R(KK),RKU(KK)
ENDDO
4 FORMAT(1X,I3,1X,F8.3,1X,F8.3,1X,F8.3)
WRITE(2,∗)‘--------------------------------’
RETURN
END
A.5. Computation of Design Flood for Medium to Small Irrigation Project: Unit Hydrograph Technique
Design of any hydrologic project including irrigation projects, requires estimation of design flood. For estimating the design flood followed by fixing the capacity of the spillway, generally three types of
design storms are used: (1) standard project storm (SPS), (2) probable maximum storm or probable maximum precipitation (PMP), and (3) frequency-based storms (FBS).
The SPS is the most severe rainstorm that has actually occurred over a watershed during the period of available records. It is used in the design of all water resources projects where much risk is not involved and economic considerations are taken into account. The SPS is used for design of medium irrigation or water resources projects.
The PMP is defined as the greatest depth of precipitation for a given period which is physically possible over a particular area and geographical location at a certain time of year. PMP is required to estimate the probable maximum flood, used in the design of spillways for large dams including major water resources or irrigation projects. The PMP can be estimated either by statistical methods or by the physical method in which historical rainstorms are maximized. It is generally determined from the greatest rainfall depth associated with severe rainstorm (i.e., SPS).
In FBS, a design flood can be estimated using flood-frequency analysis or frequency analysis of runoff obtained from the maximum rainfall–runoff analysis. The former involves a frequency analysis of long-term streamflow data at a site of interest. The latter involves either the frequency analysis of rainfall followed by rainfall–runoff analysis or frequency analysis of peak runoff estimated from maximum rainfall–peak runoff analysis.
Here, the appendix presents an SPS-based design flood estimation followed by fixing the spillway capacity. The procedure for estimating the design flood is as follows:
1. Determine the most severe rainfall storm (SPS) using the historical data. In India, it can be obtained by a written request to the India Meteorological Department (IMD) for a particular geographical location of interest.
2. Determine the short-term temporal distribution of the SPS: Using the SRRG data, average temporal distribution can be determined, and the same will be used in the determination of temporal distribution of SPS. The same may be requested from the IMD.
3. Determine the rainfall hyetograph (i.e., rainfall intensity vs. time graph and table).
4. Use the constant abstraction rate of the storm (i.e., phi-index), and determine the excess rainfall intensity followed by rainfall excess.
5. Use the derived unit hydrograph (UH) of particular duration for the given catchment.
6. Develop a critical sequence of the rainfall excess using the coordinate of the UH. For arriving at the critical sequences, the following steps are involved:
a. The ordinates of the design UH are written in a column.
b. The highest rainfall excess is written against the peak ordinate of the design UH. The second highest rainfall excess ordinate is written against the second highest ordinate of the UH, which is next to the peak ordinate, and so on. If all the ordinates of UHs are not covered by the rainfall excesses, zeros are put against them.
c. The column of the rainfall excess sequence so generated in step (b) is reversed, i.e., the last value of the rainfall excess is put as the first, the “last but one” is written at the place of the second, and so on. This arrangement of the rainfall excesses is called the “critical sequences.”
7. This critical sequenced rainfall excess is convoluted with design UH to arrive at the design flood.
Example A5.1: Estimate the design flood for a medium irrigation project having catchment area of 256 sq km corresponding to the recorded standard project storm (SPS) of 53.3 cm in 48 h. The distribution of rainfall and 2-h UH ordinates are given as under:
Time (h) | 0 | 2 | 4 | 6 | 8 | 10 | 12 | 14 | 16 | 18 | 20 | 22 | 24 |
%Rainfall | 0 | 21.0 | 30.75 | 39.5 | 45.25 | 51.5 | 56.0 | 59.75 | 64.4 | 68.0 | 71.25 | 74.5 | 77.0 |
Time (h) | 26 | 28 | 30 | 32 | 34 | 36 | 38 | 40 | 42 | 44 | 46 | 48 | |
%Rainfall | 79.25 | 81.75 | 83.75 | 86.0 | 88.0 | 90.0 | 91.5 | 93.5 | 95.25 | 97.0 | 99.0 | 100.0 | |
Time (h) | 0 | 2 | 4 | 6 | 8 | 10 | 12 | 14 | 16 | 18 |
UH (m3/s/cm) | 0 | 6.69 | 22.3 | 83.61 | 178.73 | 84.73 | 33.45 | 16.17 | 6 | 0 |
Assume phi-index value of 0.10 cm/h and base flow of 34.0 m3/s.
Solution:
(i) Temporal distribution of SPS and excess rainfall estimation
Time (h) | 48-h Rainfall Distribution (%) | Cumulative Rainfall (cm) | Incremental Rainfall (cm) | Rainfall Intensity (cm/h) | Phi-Index (cm/h) | Excess Rainfall Intensity (cm/h) | Excess Rainfall Depth (cm) |
(i) | (ii) | (iii) | (iv) | (v) | (vi) | (vii) | (viii) |
0 | 0 | 0 | 0 | 0.00 | 0.1 | 0.00 | 0.00 |
2 | 21 | 11.19 | 11.19 | 5.60 | 0.1 | 5.50 | 11.00 |
4 | 30.75 | 16.39 | 5.2 | 2.60 | 0.1 | 2.50 | 5.00 |
6 | 39.5 | 21.05 | 4.66 | 2.33 | 0.1 | 2.23 | 4.46 |
8 | 45.25 | 24.12 | 3.07 | 1.54 | 0.1 | 1.44 | 2.88 |
10 | 51.5 | 27.45 | 3.33 | 1.67 | 0.1 | 1.57 | 3.14 |
12 | 56 | 29.85 | 2.4 | 1.20 | 0.1 | 1.10 | 2.20 |
14 | 59.75 | 31.85 | 2 | 1.00 | 0.1 | 0.90 | 1.80 |
16 | 64.4 | 34.33 | 2.48 | 1.24 | 0.1 | 1.14 | 2.28 |
18 | 68 | 36.24 | 1.91 | 0.96 | 0.1 | 0.86 | 1.72 |
20 | 71.25 | 37.98 | 1.74 | 0.87 | 0.1 | 0.77 | 1.54 |
22 | 74.5 | 39.71 | 1.73 | 0.87 | 0.1 | 0.77 | 1.54 |
24 | 77 | 41.04 | 1.33 | 0.66 | 0.1 | 0.56 | 1.12 |
26 | 79.25 | 42.24 | 1.2 | 0.60 | 0.1 | 0.50 | 1.00 |
28 | 81.75 | 43.57 | 1.33 | 0.66 | 0.1 | 0.56 | 1.12 |
30 | 83.75 | 44.64 | 1.07 | 0.54 | 0.1 | 0.44 | 0.88 |
32 | 86 | 45.84 | 1.2 | 0.60 | 0.1 | 0.50 | 1.00 |
34 | 88 | 46.9 | 1.06 | 0.53 | 0.1 | 0.43 | 0.86 |
36 | 90 | 47.97 | 1.07 | 0.54 | 0.1 | 0.44 | 0.88 |
38 | 91.5 | 48.77 | 0.8 | 0.40 | 0.1 | 0.30 | 0.60 |
Table Continued |
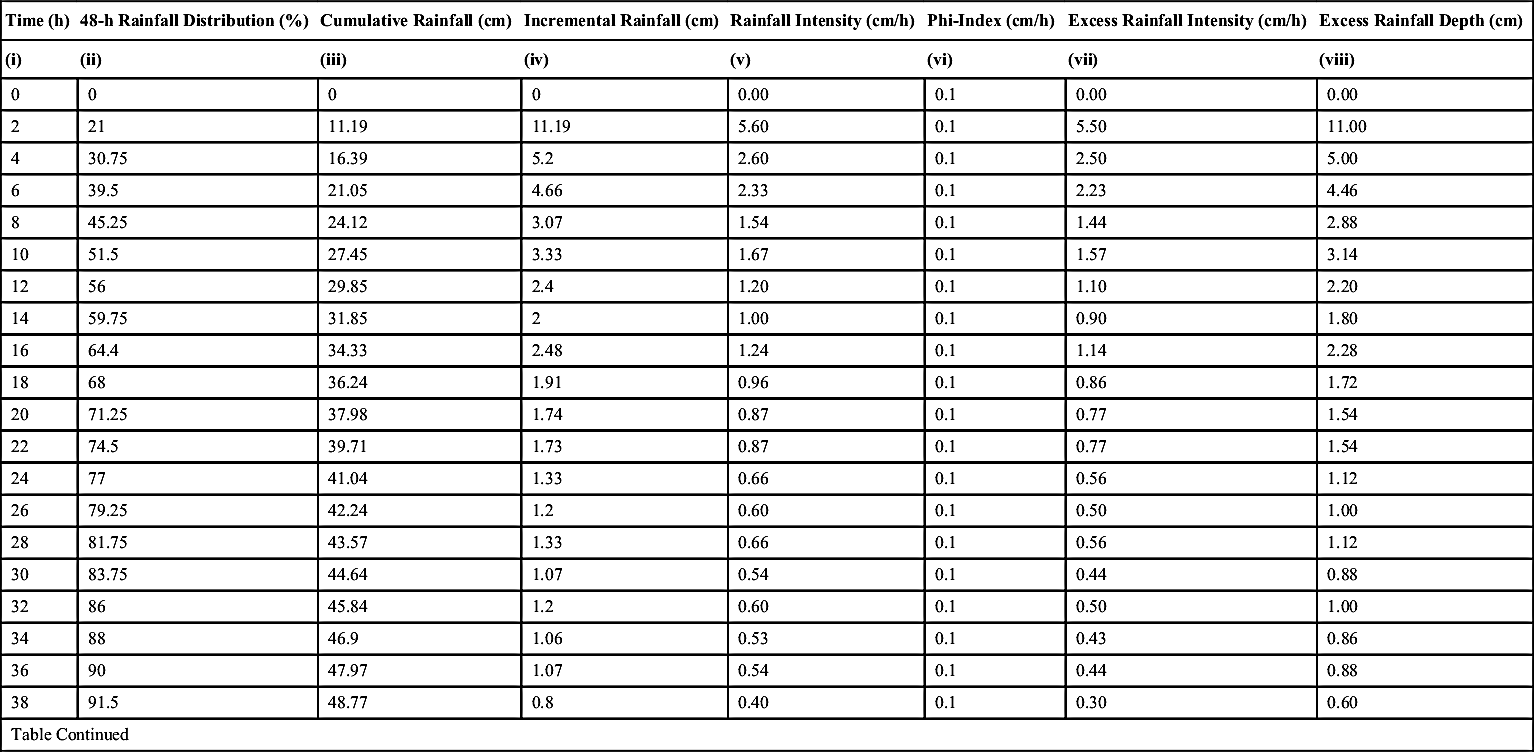
Time (h) | 48-h Rainfall Distribution (%) | Cumulative Rainfall (cm) | Incremental Rainfall (cm) | Rainfall Intensity (cm/h) | Phi-Index (cm/h) | Excess Rainfall Intensity (cm/h) | Excess Rainfall Depth (cm) |
(i) | (ii) | (iii) | (iv) | (v) | (vi) | (vii) | (viii) |
40 | 93.5 | 49.84 | 1.07 | 0.54 | 0.1 | 0.44 | 0.88 |
42 | 95.25 | 50.77 | 0.93 | 0.47 | 0.1 | 0.37 | 0.74 |
44 | 97 | 51.7 | 0.93 | 0.47 | 0.1 | 0.37 | 0.74 |
46 | 99 | 52.77 | 1.07 | 0.54 | 0.1 | 0.44 | 0.88 |
48 | 100 | 53.3 | 0.53 | 0.26 | 0.1 | 0.16 | 0.32 |
(ii) Critical sequencing of rainfall
Time (h) | 2-h UH (m3/s/cm) | Rainfall Excess (cm) | Sequencing | Critical Sequencing of Excess Rainfall (cm) |
(i) | (ii) | (iii) | (iv) | (v) |
0 | 0 | 0.00 | 0.00 | 1.8 |
2 | 6.69 | 11.00 | 2.28 | 2.2 |
4 | 22.3 | 5.00 | 2.88 | 2.34 |
6 | 83.61 | 4.46 | 4.46 | 3.14 |
8 | 178.73 | 2.88 | 11.0 | 5.0 |
10 | 84.73 | 3.14 | 5.0 | 11.0 |
12 | 33.45 | 2.20 | 3.14 | 4.46 |
14 | 16.17 | 1.80 | 2.34 | 2.88 |
16 | 6 | 2.28 | 2.2 | 2.28 |
18 | 0 | 1.72 | 1.8 | |
20 | | 1.54 | 0 | |
22 | | 1.54 | 0 | |
24 | | 2.34 | 0 | |
26 | | 1.12 | 0 | |
28 | | 0.88 | 0 | |
30 | | 1.00 | 0 | |
32 | | 0.86 | 0 | |
34 | | 0.88 | 0 | |
36 | | 0.60 | 0 | |
Table Continued |
Time (h) | 2-h UH (m3/s/cm) | Rainfall Excess (cm) | Sequencing | Critical Sequencing of Excess Rainfall (cm) |
(i) | (ii) | (iii) | (iv) | (v) |
38 | | 0.88 | 0 | |
40 | | 0.74 | 0 | |
42 | | 0.74 | 0 | |
44 | | 0.88 | 0 | |
46 | | 0.32 | 0 | |
48 | | 0.00 | 0 | |
(iii) Determination of design flood hydrograph
Time (h) | 2-h UH (m3/s/cm) | Direct Runoff From Incremental Excess Rainfall Depth (D), cm | Total DRH (m3/s) | Base Flow (m3/s) | Total Runoff (m3/s) |
D1 = 1.8 | D2 = 2.2 | D3 = 2.34 | D4 = 3.14 | D5 = 5.0 | D6 = 11.0 | D7 = 4.46 | D8 = 2.88 | D9 = 2.28 |
0 | 0 | 0.00 | | | | | | | | | 0.00 | 34.00 | 34.00 |
2 | 6.69 | 12.04 | 0.00 | | | | | | | | 12.04 | 34.00 | 46.04 |
4 | 22.3 | 40.14 | 14.72 | 0.00 | | | | | | | 54.86 | 34.00 | 88.86 |
6 | 83.61 | 150.50 | 49.06 | 15.65 | 0.00 | | | | | | 215.21 | 34.00 | 249.21 |
8 | 178.73 | 321.71 | 183.94 | 52.18 | 21.01 | 0.00 | | | | | 578.84 | 34.00 | 612.84 |
Table Continued |
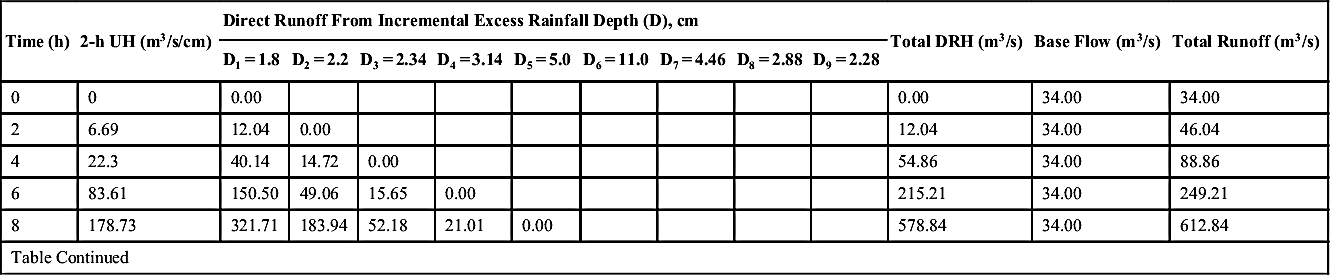
Time (h) | 2-h UH (m3/s/cm) | Direct Runoff From Incremental Excess Rainfall Depth (D), cm | Total DRH (m3/s) | Base Flow (m3/s) | Total Runoff (m3/s) |
D1 = 1.8 | D2 = 2.2 | D3 = 2.34 | D4 = 3.14 | D5 = 5.0 | D6 = 11.0 | D7 = 4.46 | D8 = 2.88 | D9 = 2.28 |
10 | 84.73 | 152.51 | 393.21 | 195.65 | 70.02 | 33.45 | 0.00 | | | | 844.84 | 34.00 | 878.84 |
12 | 33.45 | 60.21 | 186.41 | 418.23 | 262.54 | 111.50 | 73.59 | 0.00 | | | 1112.47 | 34.00 | 1146.47 |
14 | 16.17 | 29.11 | 73.59 | 198.27 | 561.21 | 418.05 | 245.30 | 29.84 | 0.00 | | 1555.36 | 34.00 | 1589.36 |
16 | 6 | 10.80 | 35.57 | 78.27 | 266.05 | 893.65 | 919.71 | 99.46 | 19.27 | 0.00 | 2322.78 | 34.00 | 2356.78 |
18 | 0 | 0.00 | 13.20 | 37.84 | 105.03 | 423.65 | 1966.03 | 372.90 | 64.22 | 15.25 | 2998.13 | 34.00 | 3032.13 |
20 | | | 0.00 | 14.04 | 50.77 | 167.25 | 932.03 | 797.14 | 240.80 | 50.84 | 2252.87 | 34.00 | 2286.87 |
22 | | | | 0.00 | 18.84 | 80.85 | 367.95 | 377.90 | 514.74 | 190.63 | 1550.91 | 34.00 | 1584.91 |
24 | | | | | 0.00 | 30.00 | 177.87 | 149.19 | 244.02 | 407.50 | 1008.58 | 34.00 | 1042.58 |
26 | | | | | | 0.00 | 66.00 | 72.12 | 96.34 | 193.18 | 427.64 | 34.00 | 461.64 |
28 | | | | | | | 0.00 | 26.76 | 46.57 | 76.27 | 149.60 | 34.00 | 183.60 |
30 | | | | | | | | 0.00 | 17.28 | 36.87 | 54.15 | 34.00 | 88.15 |
32 | | | | | | | | | 0.00 | 13.68 | 13.68 | 34.00 | 47.68 |
34 | | | | | | | | | | 0.00 | 0.00 | 34.00 | 34.00 |
36 | | | | | | | | | | | 0.00 | 34.00 | 0.00 |
38 | | | | | | | | | | | | | |
40 | | | | | | | | | | | | | |
42 | | | | | | | | | | | | | |
44 | | | | | | | | | | | | | |
46 | | | | | | | | | | | | | |
48 | | | | | | | | | | | | | |
A.6. Computation of Design Flood for Microirrigation Projects: SCS-CN Method
This method is based on the Soil Conservation Services—Curve Number (SCS-CN) technique (SCS, 1985) through which the designed value of runoff depth is estimated to correspond to design rainfall. The designed rainfall storm corresponds to the daily maximum rainfall of 25–40 years of return period. Once the designed rainfall storm is estimated, the design runoff depth can be computed using the following formula:
Rd=(Pd−λS)2Pd+(1−λ)S
(A6.1)where
Rd is the design value of runoff depth (mm),
Pd is the design rainfall depth (mm),
S is the maximum potential abstraction (mm), and
λ is the abstraction coefficient. The value of
λ ranges between 0.1 and 0.3. For black soil,
λ =
0.1 for antecedent moisture condition (AMC)-II- and AMC-III-type conditions, and
λ =
0.3 for AMC I condition. Since parameter
S, i.e., maximum potential abstraction, can vary in the range of 0
≤
S ≤
∞, it is mapped onto a dimensionless curve number (
CN), varying in a more appealing range 0
≤
CN ≤
100, as follows:
S=25400CN−254
(A6.2)Although
CN theoretically varies from 0 to 100, the practical design values validated by experience lie in the range (40, 98). The value of
CN tabulated in
Appendix A.8 corresponds to the hydrological soil group, AMC condition, and land use.
Once the value of
Rd is determined from
Eq. (A6.1), the peak discharge or designed discharge can be estimated using the following relationship:
Qp=2.08×A×Rdtp
(A6.3)where Qp is the designed discharge (m3/s), A is the catchment area (sq km), Rd is the design runoff depth (cm), and tp is the time to peak of the catchment (h).
The value of tp for small catchment can be determined using the following formula:
tp=0.6tc+t0.5c
(A6.4)where tp is the time to peak (h) and tc is the time of concentration (h). The value of tc can be determined by using the Kirpich formula:
tc=0.01947L0.77S−0.385
(A6.5)where tc is in min, L is the length of the channel from headwater to the outlet (m), and S is the slope (m/m).
Another formula for relating the peak discharge rate with the runoff depth and time to peak, generally used in southern India is:
Qp=1.46×A×Rdtp
(A6.6)The value of tp (h) can be determined by using the following relationships:
tp=0.76A0.28;L/W>4tp=0.48A0.28;L/W<4
(A6.7)where
L is the length of the watershed and
W is the average width of the watershed (m).
Example A6.1: Estimate the design discharge for a microirrigation project having a catchment area of 25 sq km with a length:width (L:W) ratio of 6. The daily maximum rainfall for 25 years return period is 150 mm. The soil group of the catchment is red soil. The land uses are:
Cultivated land with crops is 60% and waste land is 40%.
Solution:
Catchment area, A = 25 sq km; L:W = 5
Hydrological soil group = red soil (Group B)
(i) The value of
tp can be determined using
Eq. (A6.6) as:
tp=0.76A0.28=0.76×250.28=1.87h
(ii) Computation of CN for hydrological soil group B:
S. No. | Land Uses | %Area | CN | Weighted CN |
1 | Cultivated land with crops | 60.0 | 75 | 45 |
2 | Waste land | 40.0 | 80 | 32 |
| | 100 | Weighted CN | 77 |
The value of maximum potential abstraction, S will be:
S=25400CN−254=2540077−254=75.87mm
(iii) Determined design runoff depth for Pd = 150 mm assuming λ = 0.3
Rd=(Pd−λS)2Pd+(1−λ)S=(150−0.3×75.87)2150+0.7×75.87=79.71mm=7.97cm
(iv) Determine the designed discharge using
Eq. (A6.3)Qp=2.08×A×Rdtp=2.08×25×7.971.87=221.6m3/s