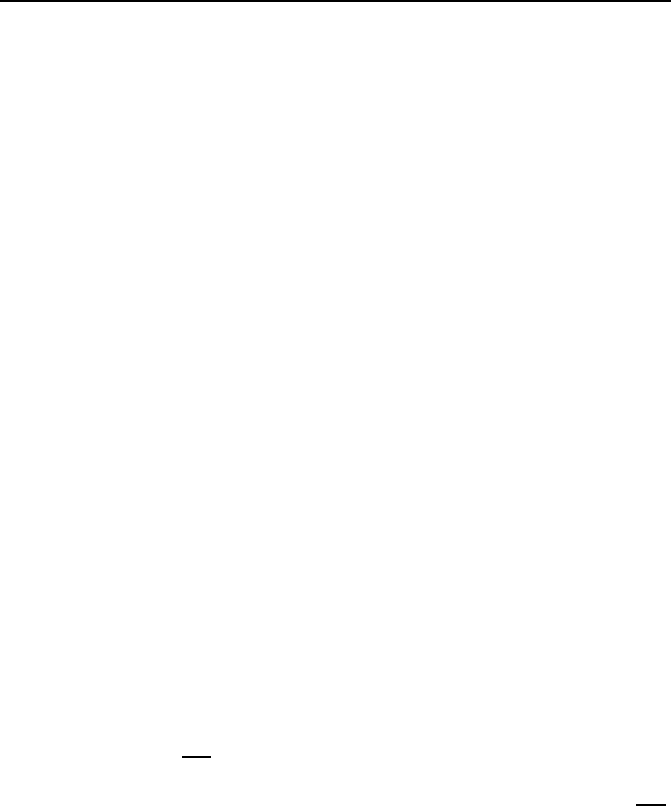
Super-Regenerative Detection 233
Recently, a metamateria l-based resonator has been explored in [254] to
improve the Q with compact area at the mm-wave frequency region. A split-
ring-res onator (SRR) can be des igned in the CMOS proces s top-metal layer
to provide nega tive permeability (µ) for mm-wave propagation. When loading
SRR to a host transmission-line (TL-SRR), the integrated structure becomes
a non-transmission medium with s ingle-negative property (µ · ε < 0) in the
vicinity of re sonance frequency. A sharp stop-band is thereby formed such
that the incident mm-wave can be perfectly reflected at SRR load with a
stable sta nding-wave esta blished in the host T-line [254]. Compared to the
traditional L C -tank-based resonator, TL-SRR has stable EM-energy storage
within a compact area, which results in a much higher Q factor. As such,
it becomes relevant to study the CMOS on-chip SRR for the compact and
high-sensitivity SRX design of the THz imager.
In this chapter, firstly, the design of on-chip metamaterial resonators is
explored based on differ ential T-line loaded with SRR and CSRR beyond 70
GHz. As a demonstration of the idea, two oscillators based on SRR and CSRR
resonators are implemented with 65nm CMOS process at 76 GHz and 96 GHz,
respectively. The s tate-of-the-art performance shows that the phase noise and
FOM of SRR achieves -108.8 dBc/Hz and -182.1 dBc/Hz (@10 MHz offset),
respectively. The power is reduced dramatically to 2.7 mW compared to the
existing designs on SWOs [246, 238, 239]. And the CSRR oscillator shows
a state-of-the-art pha se noise of -111.5 dBc/Hz (@10 -MHz offset) and FOM
of -182.4 dBc/Hz. Secondly, two super-regenerative receivers with quench-
controlled metamaterial high-Q oscillators by TL-C SRR and TL-SRR are
demonstrated with improved sensitivity over traditional LC-tank resona tor-
based designs at 96 GHz and 135 GHz, respectively. With a sharp stop-ba nd
introduced by the metamater ial resonators, high-Q oscillato ry a mplifications
are a chieved. The 96-GHz DTL-CSRR-based SRX has a compact core chip
area of 0.014 mm
2
, and it is measured with power consumption of 2.8 mW,
sensitivity of -79 dBm, noise figure (NF) of 8.5 dB, and noise equivalent power
(NEP) of 0.67 fW/
√
Hz. T he 135-GHz DTL-SRR-based SRX has a compact
core chip area of 0.0085 mm
2
, and it is measured with power consumption of
6.2 mW, sensitivity of -76.8 dBm, NF of 9.7 dB, and NEP of 0.9 fW/
√
Hz.
The proposed SRXs have 2 .8-4 dB sensitivity improvement a nd 6 0% smaller
core chip are a when compared to the conventional SRX with LC-ta nk-based
resonator at similar fre quencies.
10.2 Fundamentals of Super-Regenerat ive
Amplification
Generally, a SRX consists of a quench-controlled oscillator injected by an
external sig nal and an envelope detector. The process of injecting an external
signal into a quench-controlled oscillator is firstly reviewed to under stand the
operation of SRX, called super-regener ative amplification (SRA).